Research Report 3: Phage Clearance
- BJW
- Sep 2, 2019
- 6 min read
Updated: Apr 28, 2020
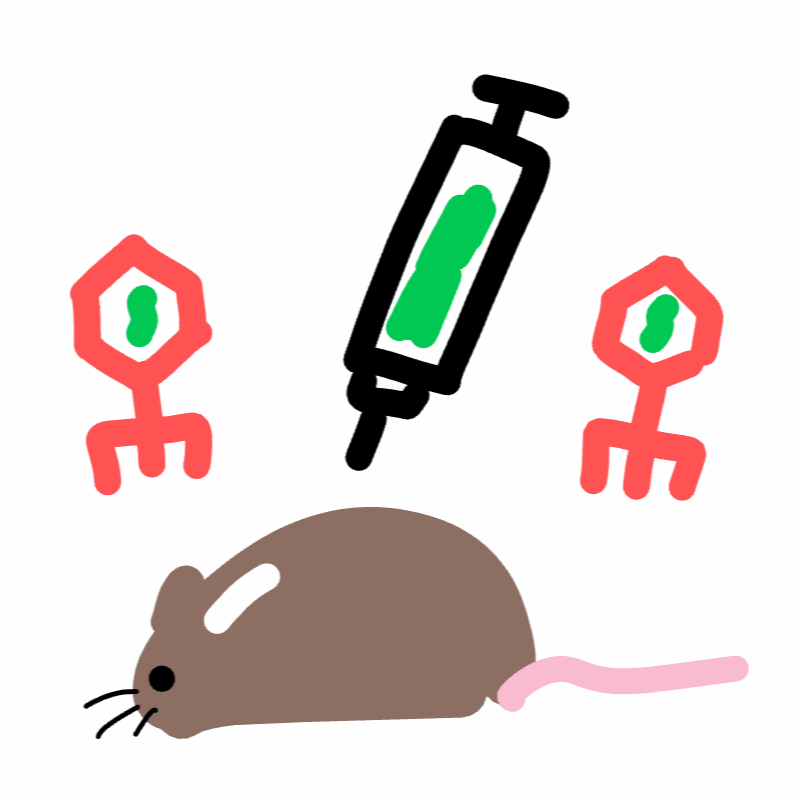
Silly mouse, phages are for humans!
While phage therapy initially seemed like a perfect solution, I think I've found one issue that will have to be resolved before phage therapy can become effective.
I’ll cut to the chase. There’s a particular type of cell in our bodies, called liver sinusoidal endothelial cells or LSECs. As the name might suggest, these cells are based in the liver. Along with another type of cells called Kupffer cells (cute name), LSECs remove particulate matter and detritus from the blood [1,2].
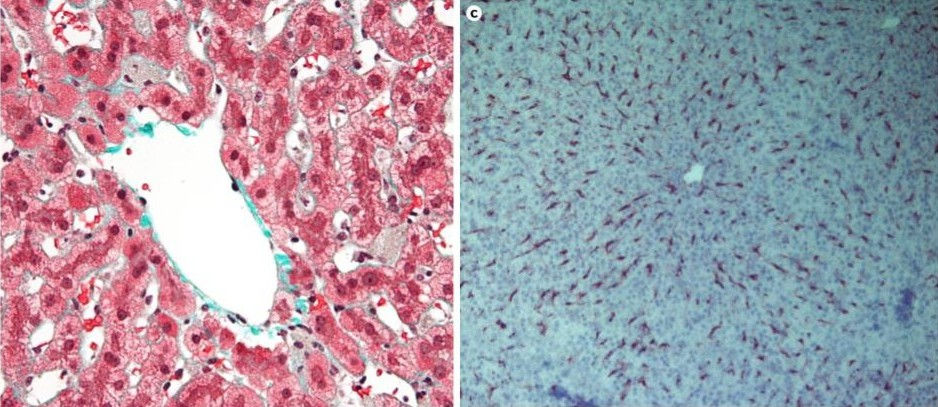
Kupffer cells (L) and LSECs (R). The dark objects in the right picture are stained LSECs
Source: Wikipedia, [3]
Viruses are among the biological flotsam cleaned up by these cells, though it’s the LSECs that clean up viruses mainly. As Dr. Ganesen of Ohio State University said in a 2011 paper: “The phenomenon [of viral clearance] has been described for nearly all viruses tested; exceptions are rare.” Most problematically, experiments with rabbits and rats showed that bacteriophages are no exception. Ganesan and co also put forth the troubling statistic that half-lives of introduced viruses range from 2-4 minutes [2]. A chart pulled from Dr. Ganesen’s paper illustrates how rapidly viruses are cleared.
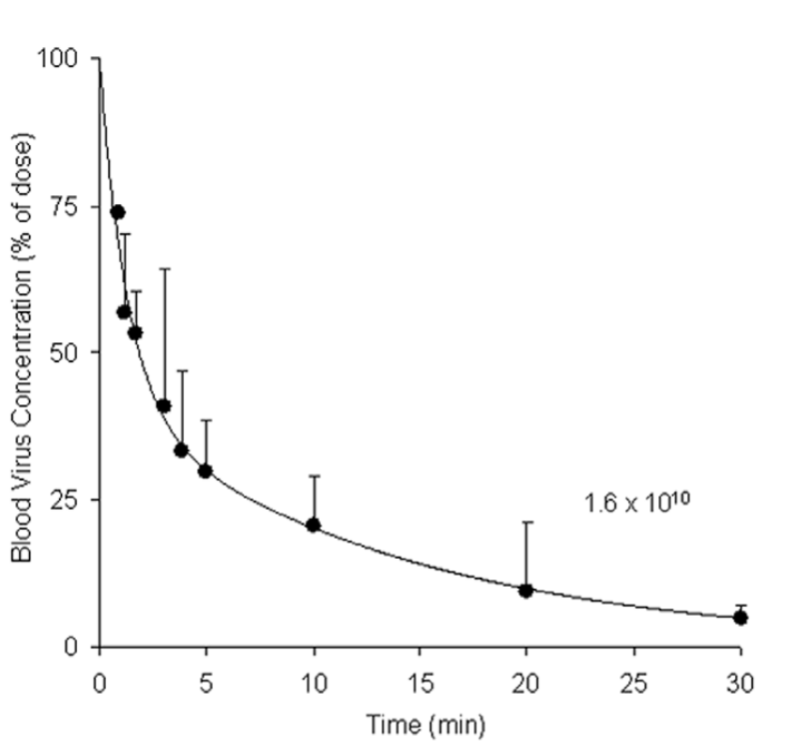
Bloodstream concentration of virus in rats (adenovirus in this case) over time [2]
Such rapid clearance is pretty bad news for the reliable application of phage therapy. Less phage, less bacteria-killing and worse clinical outcomes. Not good.
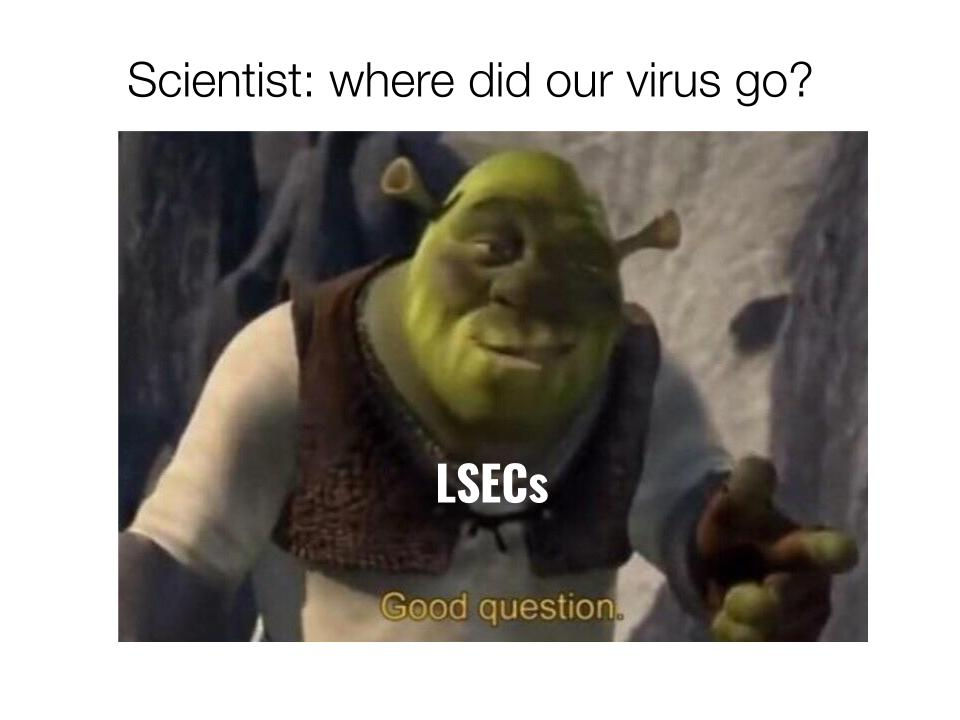
However, this isn’t an insurmountable problem.
Goodridge et al. (“et alia,” or “and others) in a 2010 paper describe several potential solutions to the issue of LSEC virus removal. Let’s walk through one of these solutions.
Serial Injection and “Natural Selection”
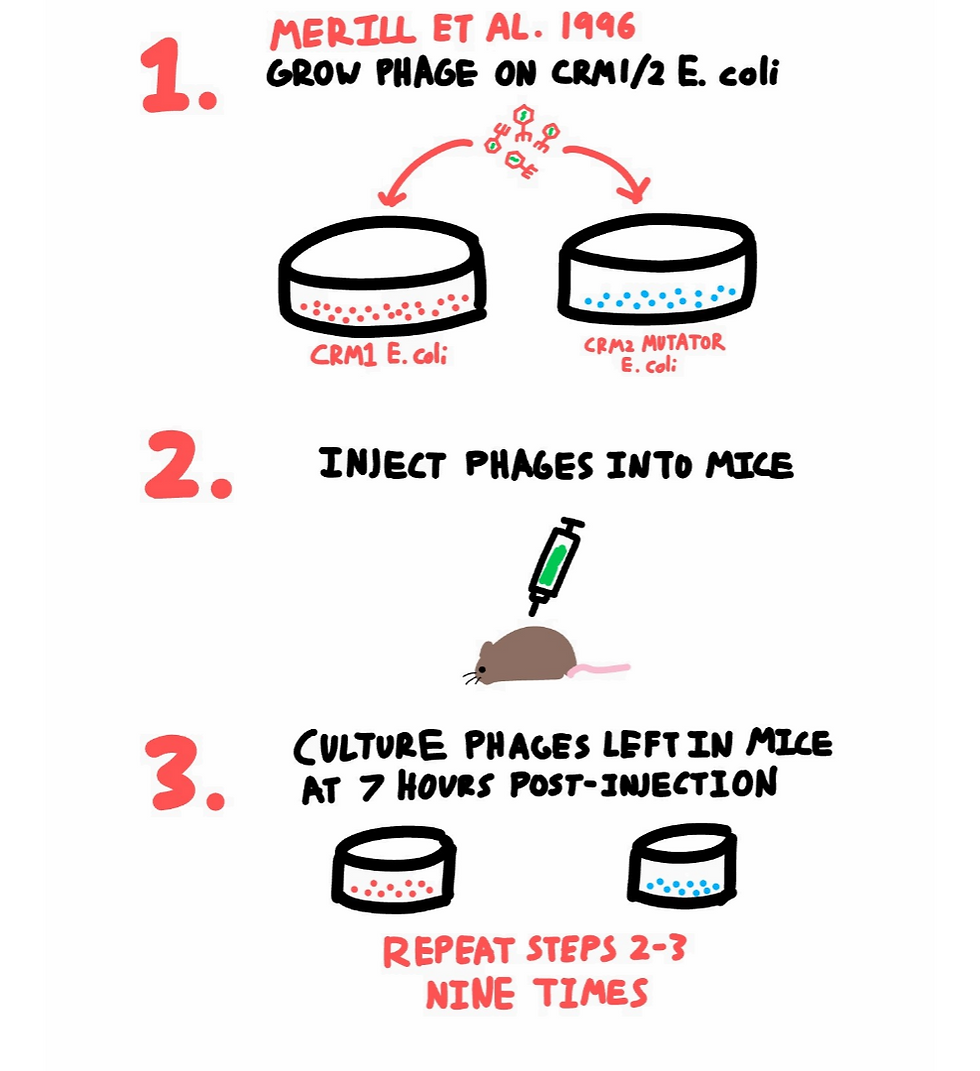
Merril et al. 1996 had a pretty straight-forward solution. Instead of actively trying to design a bacteriophage with longer circulation times, they decided to let natural selection do the work for them.
So basically...
Merril et al. took some phage, stuck it inside a mouse, waited 7 hours, then extracted the phages that hadn’t been filtered out. They cultured more of these extracted phages, then injected it into another mouse. Rinse and repeat. They did this culture-injection cycle 10 times total, each time with the product phage of the previous step.
Let me explain in a little more detail now.
Merril and co grew their phage on two different strains of E. coli (see diagram). One was the CRM1 strain, completely normal and unmodified E. coli. The second strain, CRM2 E. coli, was special. It was something called a “mutator strain.” The phages grown using this strain would have a higher rate of mutation. In theory, this means that the phages grown using the mutator E. coli would have higher diversity than the ones grown using vanilla E. coli. It was hoped that these mutated phages would possess more characteristics allowing them to evade the LSECs (possibly better) than the vanilla phages.
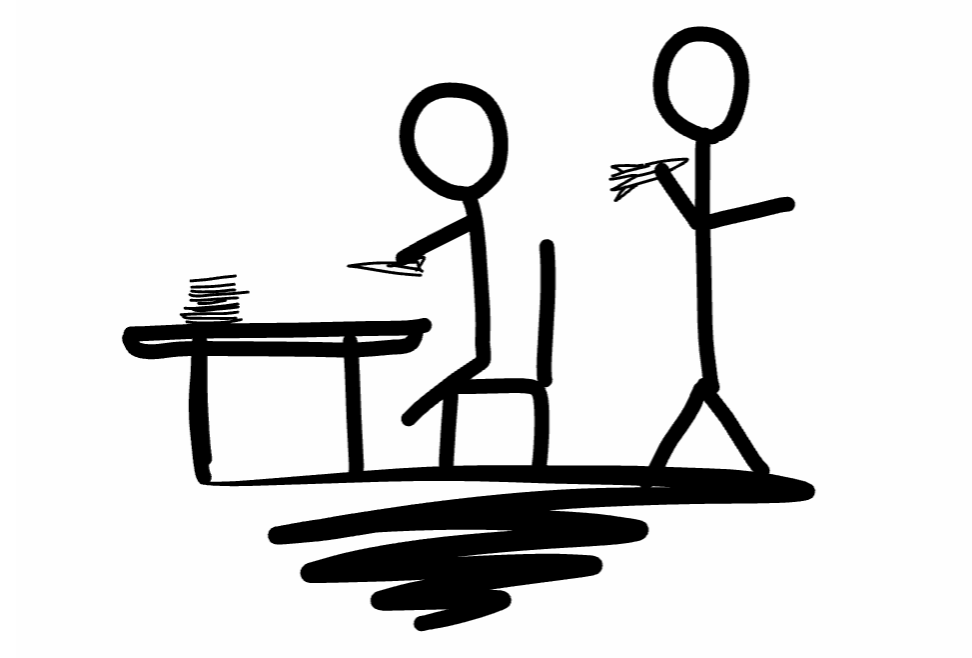
Meet Bob and Steve
Let me explain why mutator E. coli was used in another way. Say there are two people, Steve and Bob, who are folding paper airplanes. The template the paper airplanes are being made from is kind of sucky, they don’t produce very far-flying planes. Steve makes paper airplanes that are mostly identical to the template, with some every now and then having some variation in folds. Bob, on the other hand, has a bad memory, he keeps folding paper airplanes with more and more variation as time goes on. When Steve and Bob try flying their airplanes, they get very different results. Steve’s airplanes fly consistently, but suckily. Some of Bob’s airplanes fly atrociously, a ball of paper would have been a better design. However, others fly amazingly, far outsoaring the rest. Steve folding airplanes represents the normal E. coli making phages. Likewise, Bob represents the mutator E. coli.
This is why the mutator E. coli was used. The resulting mutation-induced variation does not ensure that all phages produced will perform excellently, in fact, it means quite a few will probably do far worse than the normal E. coli produced phage. You know, since mutation is a random process and all. However, it also means that there probably will be some phages that greatly outperform the normal phages.

Changes in capsid (virus capsule) proteins were found to be the
major difference between selected and unselected (starting phage)
Anyhow, the phages grown off of CRM1 and the mutator CRM2 E. coli would then be injected into their own mouse (one mouse for the normal CRM1 E. coli phage, another for the CRM2 mutator E. coli phage). Note that there weren’t any E. coli inside the mice for the phages to grow on. The phages were just simply passively floating around the mice, with the mice LSECs doing their best to remove the phages. After 7 hours, the remaining phages would be removed from the mouse and isolated.
The idea was, these remaining phages would be the ones with the LSEC-evading characteristics, as after all, they had successfully avoided getting filtered out for the 7 hours. These phages went through the cycle of culture and injection 9 more times to further select for phages able to circulate longer.
Note of clarification...
From the normal E. coli phages, note that we're not necessarily creating new and better phages, we're simply eliminating the worse phages among the starting population, and leaving the best. Classic natural selection. It's a smidgen different with the mutator E. coli phages, as there's a good chance new variations of phages are being created, with the best of these, again, being selected.
The results strongly suggested that this method of creating long-circulating phages was successful!
Gaze upon this chart here.

Merill et al. 1996 [4]
Above is a chart detailing the measured number of “plaque-forming units” (PFUs) on a log scale. A plaque-forming unit is just an entity that can cause an infection, whether it be an isolated phage, or a phage-infected bacterium that cause other bacteria to become infected. The significance of the log scale is that for every notch up or down the y-axis (6 to 7, for example), there is a 10-fold change in value. And the x-axis is just time in hours, as the label says.
Looking at the actual contents of the chart, you can see that there is a huge difference between the rates of the starter and modified phages. Due to the nature of the experiment, with shorter-circulating phages being eliminated (see explanation figure), this is no big surprise. As an added plus, Merill informs us that these end result phages are also more effective as treatment. Mice injected with the end result phages displayed less symptoms of illness compared to mice injected with the starter phages. The reason why would probably have to do with the end result phages’ longer circulation time. With more time to kill bacteria, phages would allow for a less severe illness.
What was wildly contrary to my expectations, however, was the fact that the CRM1 (normal) E. coli phages were able to circulate longer than the mutator E. coli phages. With the probable larger variation among the mutator E. coli phages, I would’ve expected that at least some would have had a mutation that improved their performance beyond that of the normal phages. Perhaps the best of the normal E. coli phages (the ones that survived the selection process) simply had the best possible characteristics and thus couldn’t be surpassed by mutation. I don’t know, that’s just speculation on my part.
It’s definitely not speculation though that this method of “serial injection” produces phage not as direly affected by the issue of LSEC virus clearance. So besides economic considerations (phages can’t be patented, so no money for big pharma), I’m still baffled why phage therapy isn’t a thing yet. Maybe the economics stuff is all there is to it, maybe I’m just wasting my time. I don’t know, I’m no economist, I can’t say.
Thanks for reading!
- BJW
Source Literature
3. Shetty S, Lalor PF, Adams DH. Liver sinusoidal endothelial cells — gatekeepers of hepatic immunity [Internet]. Nature Reviews Gastroenterology & Hepatology. 2018. pp. 555–567. doi:10.1038/s41575-018-0020-y
Comentarios